2024
From the lab of Dae-sik Lim, KAIST, Republic of Korea
Used:
R-Spondin 1 (Qk006)
From the lab of Daniel J. Fazakerley, University of Cambridge
From the lab of Marta Shahbazi, MRC Laboratory of Molecular Biology, Cambridge
Used:
Gremlin 1 (Qk015)
From the lab of Kirmo Wartiovaara, University of Helsinki
Used:
Activin A (Qk001)
From the lab of Florian Merkle, University of Cambridge
Used:
Human BDNF (Qk050)
From the lab of Jose Silva, Guangzhou, China
Used:
Mouse LIF (Qk018)
From the lab of Jan Żylicz, University of Copenhagen, Denmark
From the lab of Tomasz Nowakowski, University of California, San Francisco
Used:
TGF-β3 (Qk054)
From the lab of Margareta Wilhelm, Karolinska Institutet
Used:
FGF2-G3 (Qk053)
From the lab of José Silva, Guangzhou Laboratory
From the lab of Magdalena Zernicka-Goetz, University of Cambridge
Used:
Activin A (Qk001)
From the lab of Dannielle Engle, Salk Institute for Biological Studies
From the lab of Robert Thomas, Loughborough University
From the lab of Stephen O’Rahilly, University of Cambridge
Used:
GDF-15 (Qk017)
2023
From the lab of Ivana Barbaric, University of Sheffield
Used:
FGF2-G3 (Qk053)
From the lab of Elena Carbognin and Graziano Martello, University of Padua
From the lab of Karl Wahlin, University of California San Diego
From the lab of Siim Pauklin, University of Oxford
Used:
Activin A (Qk001)
From the lab of Luis M. Escudero, University of Sevilla
Used:
LIF (Qk018)
From the labs of Catherine H. Wilson, University of Cambridge and James E. Hudson, QIMR Berghofer Medical Research Institute
From the labs of Austin Smith, University of Cambridge and Kevin J. Chalut, University of Exeter
Used:
hLIF (Qk036)
From the labs of John C. Marioni, European Bioinformatics Institute and Florian T. Merkle, University of Cambridge
Used:
BDNF (Qk050)
From the labs of James Briscoe and Elly M. Tanaka, Francis Crick Institute and the Research Institute of Molecular Pathology (IMP) of Vienna
Used:
Murine LIF (Qk018)
From the lab of Graziano Martello and Francesco Argenton, University of Padova
Used:
hLIF (Qk036)
From the lab of Graziano Martello, University of Padova
From the lab of Magdalena Zernicka-Goetz, University of Cambridge
Used:
Activin A (Qk001)
From the lab of Magdalena Zernicka-Goetz, University of Cambridge
Used:
Activin A (Qk001)
From the lab of Paul W. Burridge, Northwestern University Feinberg School of Medicine
Used:
TGFβ3 (Qk054)
From the lab of Sun-Young Kong, National Cancer Center, Korea
Used:
R-spondin-1 (Qk006)
From the lab of Karl Wahlin, University of California San Diego
From the labs of David A. Turner, University of Liverpool and Ben Steventon, University of Cambridge
Used:
Murine LIF (Qk018)
From the labs of Emmanuel Contassot and Alexander A. Navarini, University of Basel
From the labs of Tamir Rashid, Imperial College London and Eileen Gentleman, King’s College London.
Used:
Activin A (Qk001)
From the lab of Stephen O’Rahilly, University of Cambridge, UK and Nicholas Mancuso, University of Southern California, USA.
Used:
Human GDF-15 protein (Qk017)
From the labs of Jamie A. Hackett, European Molecular Biology Laboratory EMBL-Rome, Davide Cacchiarelli, Telethon Institute of Genetics and Medicine and Graziano Martello, University of Padua.
From the lab of Anthony Davenport, University of Cambridge
Used:
Zebrafish FGF-2 protein (Qk002)
From the lab of Nicolas Rivron, IMBA, Austrian Academy of Sciences
Used:
Human LIF protein (Qk036)
2022
From the lab of Stephen O’Rahilly, University of Cambridge
Used:
TGF-β growth factors, including GDF-15 (Qk017)
From the lab of Timo Otonkoski, University of Helsinki
Used:
Activin A (Qk001)
From the lab of Jorge Ferrer, Centre for Genomic Regulation (CRG)
Used:
Activin A (Qk001)
From the lab of Magdalena Zernicka-Goetz, University of Cambridge
Used:
Activin A (Qk001)
From the lab of Santiago Vernia, LMS London Institute of Medical Sciences
Used:
Activin A (Qk001)
From the lab of Alexi Savchenko, University of Toronto
Used:
TGF-β1 (Qk010)
From the lab of Daniel J. Fazakerley, University of Cambridge
Used:
FGF-2 (Qk002)
From the lab of Cristina Pina, Brunel University
Used:
Activin A (Qk001)
From the lab of Jorge Ferrer, Centre for Genomic Regulation (CRG)
Used:
Activin A (Qk001)
From the lab of Thorsten E. Boroviak, University of Cambridge
Used:
Noggin (Qk034)
From the lab of Tom Burdon, University of Edinburgh
From the lab of Elisabetta Ferretti, University of Copenhagen
From the lab of Dr Christopher D. Spicer, University of York
From the lab of Francesco Argenton, University of Padova, Italy.
Used:
Human LIF protein (Qk036)
From the lab of Ludovic Vallier, University of Cambridge
Used:
FGF-2 / bFGF 154 aa (Qk027)
2021
Luo, L, et al. ‘Hydrostatic Pressure Promotes Chondrogenic Differentiation and Microvesicle Release from Human Embryonic and Bone Marrow Stem Cells’. Biotechnology Journal (2021)
From the lab of Alicia El Haj, University of Birmingham
Huang, T et al. ‘Sex-Specific Chromatin Remodelling Safeguards Transcription in Germ Cells’. Nature, 600, 737–742 (2021)
From the lab of Petra Hajkova, MRC London Institute of Medical Sciences
Used:
BMP-2 (Qk007)
Cimino, I. et al. ‘Activation of the hypothalamic–pituitary–adrenal axis by exogenous and endogenous GDF15’. PNAS, 118, 27 (2021).
From the lab of Stephen O’Rahilly, University of Cambridge
Used:
GDF-15 (Qk017)
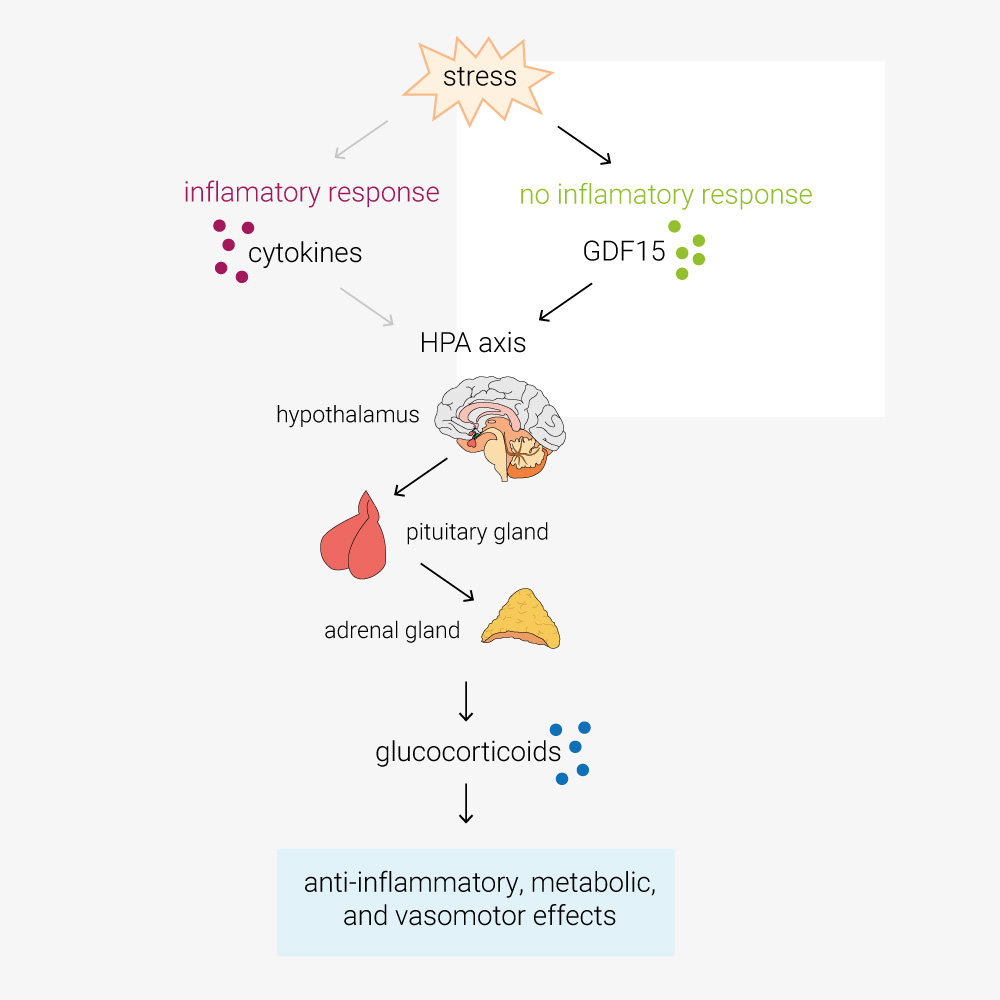
In this paper, Cinimo et al. explore the role of the TGFβ-family protein GDF15 in activation of the hypothalamic–pituitary–adrenal (HPA) axis. During infection, cytokines such as TNFα/β, IL-1 and IL-6, activate the HPA axis. This increases circulating glucocorticoids, which have anti-inflammatory, metabolic, and vasomotor effects. However, O’Rahilly lab have determined that in response to stimuli such as toxins, which don’t provoke an inflammatory response, the primary activator of the HPA axis is GDF15. GDF15 is an intriguing protein also being explored as an anti-obesity therapeutic target, these findings may have a pivotal impact on future clinical study design and open new avenues of investigation. Certainly cool science!
DOI: doi: 10.1038/s42003-021-02453-y.
From the lab of Anthony Davenport, University of Cambridge
Used:
zFGF-2 / bFGF (Qk002)
From the lab of Austin Smith, University of Cambridge & University of Exeter
In the study of embryonic stem cells, stem cells representative of naïve and primed pluripotency have been well established in the forms of embryonic stem cells (ESCs) and epiblast-derived stem cells (EpiSCs). In this study Kinoshita et al. fill the gap between early and late pluripotency in describing an intermediate state; formative stem (FS) cells. FS cells differ from both ESCs and EpiSCs, a difference beautifully exemplified by their relative contribution to chimeras. Compared with ESCs, which readily contribute to chimeras, FS chimera contribution is less frequent, and their contribution is less evenly distributed. EpiSCs on the other hand do not generally contribute to chimeras at all. FS cells were established by culturing E5.5 epiblasts, or ES cells, in N2B27 media supplemented with a low dose of Qkine Activin A alongside a Wnt inhibitor and pan-retinoic acid receptor inverse agonist. We are proud our growth factors could be part of such an exciting finding!
Masaki Kinoshita, first author, MRC Cambridge Stem Cell Institute, University of Cambridge, says:
“Formative” pluripotency exists transiently in early development and naive mouse ES cell differentiation, which cells directly respond to differentiation signals. This paper showed that formative pluripotency is now captured in culture and expands its knowledge including chimaera competency of early embryonic cells.
From the lab of Stina Simonsson, University of Gothenburg
Used:
Activin A (Qk001)
In embryonic development, growth factors are delivered in a highly controlled and targeted manner, however when differentiating iPSCs the real challenge is to effectively mimic these conditions. Consequently, iPSC differentiation is plagued by issues such as low efficiency and a lack of homogeneity. In their recent paper Andreasson et al. take a step towards improving the differentiation of iPSCs to definitive endoderm. The group employs gold nanoparticles to generate a gradient of immobilised Activin A – a member of the TGF-β superfamily that plays a key role in definitive endoderm development. Using this gradient, the group was able to deliver Activin A in a controlled and localised manor, resulting in more efficient differentiation. By deploying their innovative approach, the group observed a dose dependent response of the cells to Activin A, as defined by expression of differentiation markers SOX17 and GATA4. Their results indicate that it may be possible to define an optimal density of Activin A for definitive endoderm differentiation – a finding that could improve the homogeneity and speed of differentiation. This innovative study is a wonderful example of how reconsidering the way in which growth factors are delivered can lead to advances in our understanding of the precise control of stem cell differentiation and how these cells undertake their fate decisions.
2020
From the lab of Harry Leitch, Imperial College London
Used:
mouse LIF (Qk018)
From the lab of Graziano Martello, University of Padua
From the lab of Harry Leitch, Imperial College London
Used:
Activin A (Qk001)
2019
Reviewers comments available to view: Stadtfeld, M. Evaluation of Stuart et al.: Distinct Molecular Trajectories Converge to Induce Naive Pluripotency. Cell Stem Cell 25, 297–298 (2019). doi: 10.1016/j.stem.2019.08.009
From the lab of José Silva, University of Cambridge
2018
DOI: 10.1002/sctm.18-0084
From the lab of Tamir Rashid, Kings College London
Used:
Activin A (Qk001)