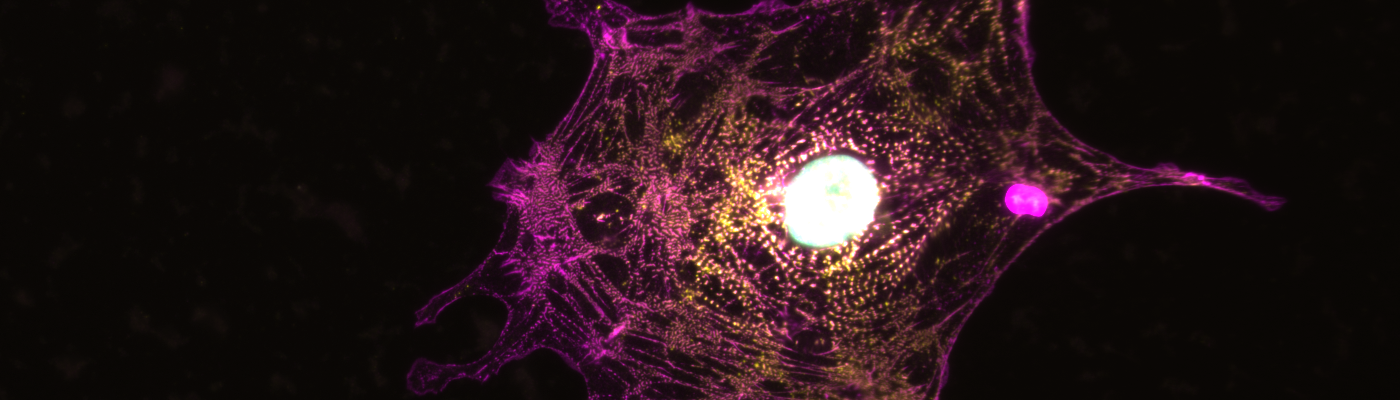
Image credit: Dr Alessandro Bertero
Cardiac organoids – the future of drug discovery?
Despite advances in research, around 90% of drugs fail during phase 1 clinical trials. New drugs for the treatment of cardiovascular disease and oncology have a particularly high failure rate, largely attributed to cardiotoxicity. Current animal models and cell-based assays do not provide accurate models for the early detection of cardiotoxicity. Cardiac organoids, sometimes referred to as cardioids are 3D cell structures that better mimic the native structure, function, and behaviour of heart tissue. Derived from stem cells, and often comprising multiple cell types, they are a powerful tool for studying and understanding cardiac development and physiology.
They are also useful in the study of a variety of diseases, including cardiovascular diseases, genetic disorders, and cancer, where they provide a more accurate representation of cardiac physiology than traditional cell-culture models by better replicating the complex tissue microenvironment and cell-cell interactions of the heart. Importantly, these 3D cell culture models have the potential to revolutionize the drug discovery process by providing more reliable and efficient methods of detecting potential cardiotoxicity during screening in early preclinical drug discovery.
Outside of drug discovery, cardiac organoids have many other applications such as regenerative medicine to generate functional cardiac tissue as a future alternative to transplantation. Additionally, cardiac organoids can be used to study the effects of aging on cardiac physiology, and on the ability of stem cells to regenerate cardiac tissue.
Although recent advances in cardiomyocyte differentiation from human induced pluripotent stem cells (iPSCs) and mesenchymal stem cells (MSCs) have enabled the formation of cardiomyocyte-containing cardiac organoids, there are several important questions to address to fully develop the potential of organoids for cardiac disease modeling and drug discovery.
- How can we improve reproducibility, throughput, and compatibility with automated high-content screening platforms and real-time functional assays?
- Can we generate enhanced models by establishing vascularization and perfusion to model complex physiology and pathology?
- A major limitation of cardiac organoids is the maturity of the cell types: how can we enhance cell maturity in cardiac organoids and other models such as spheroids and organ-on-chip models of cardiac tissues, to replicate the full range of cardiac functions and tissue-specific physiology more closely?
Building homogeneity and reproducibility into cardiac organoid production
The reproducibility of 3D cardiac models poses a challenge that must be addressed to ensure the full applicability to high- or mid-throughput screening platforms. To this end, rigorous standardization and optimization of iPSC and MSC differentiation protocols, and organoid or spheroid generation procedures, are essential. For full integration into drug discovery platforms, either during target discovery or pre-clinical toxicology screening, technologies must be developed to improve compatibility with automated handling, high-content imaging, and real-time assay platforms. In complex 3D cell culture models, parameters such as organoid size, complexity, and maturity are challenging to control and synchronize across long-term cultures.
An important trend supporting scale-up and improved reproducibility in organoid culture is the move to serum-free, chemically defined cell culture media to remove sources of inter- and intra-laboratory variability. In addition, the removal of animal-derived components and particularly growth factors or cytokines derived from mammalian cell culture, such as BMP-4, is an important step in scale-up to reduce cost whilst improving reproducibility. TGF beta family proteins such as BMP-4, are notoriously difficult to produce in a highly pure form from mammalian protein production platforms, such as CHO and NSO cells, this can lead to differences between suppliers and lots from the same supplier. Additionally, media development and refinement, including the correct selection of components and optimization of concentrations is necessary to ensure reproducible results in organoid cell culture.
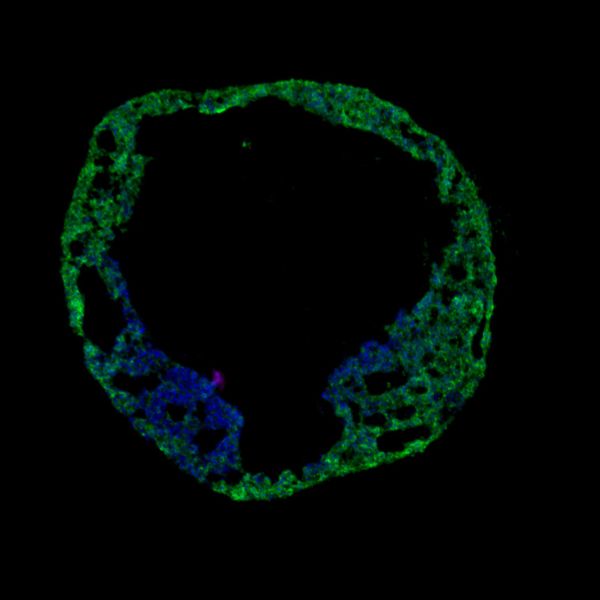
For those cardiac organoid models that rely on Matrigel, there is a drive to replace this with synthetic hydrogels, or purified recombinant extracellular matrix components, such as vitronectin. This addresses both supply chain challenges that limit scale-up, remove animal-derived components with inherent variability from the cell culture system, and importantly improve compatibility with automated cell culture. Replacement of Matrigel (or similar matrices) with synthetic hydrogels is proving an industry-wide challenge with few widely adopted solutions to date.
Automation of organoid culture and functional bioassays are key areas being addressed in order to scale organoid cell culture systems, as they play a critical role in achieving consistent organoid cultures and high-quality data. Automating laboratory processes such as sample preparation, incubation and imaging can help increase the reproducibility of the culture system while decreasing labor costs. Significant advances are being made to deliver successful scale-up of organoid cultures, and there is growing interest in overcoming remaining barriers to fully-defined 3D-cell culture systems.
Modeling vascularization in organoid culture
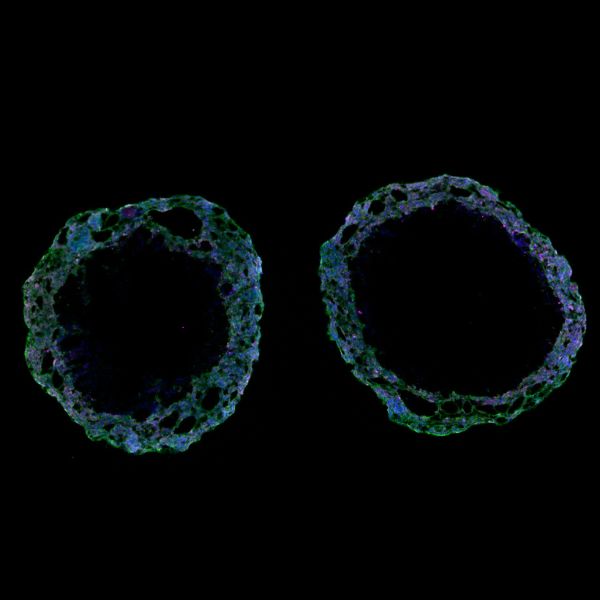
Advances in tissue engineering are providing opportunities to build more complex models of the human heart. As the complexity and size of organoid models increase, the need for a form of vascularisation to provide nutrients to these models is an area of active research. Several recent technological advances, including the use of microfluidics and micropatterning techniques, have allowed researchers to build more physiologically relevant complex models of heart tissues. This may improve the ability to reproduce complex aspects of cardiotoxicity and disease. Recently, micro vascularized cardiac organoids have been successfully generated, and particularly intriguing, is recent work on blood vessel organoids comprising self-organizing endothelial cells. The vascular lineage induction was achieved by modulating bone morphogenetic protein 4 signaling (BMP4), vascular endothelial growth factor A (VEGF 165), and fibroblast growth factor 2 (FGF-2) levels. Blood vessel capillary networks formed by the self-organization of endothelial cells and pericytes present a fascinating avenue for further development of organoid models. Although of intense basic research interest, these complex models may have limited application in high throughput studies necessary for efficient new drug discovery. A simple and reproducible blood vessel organoid model however may open opportunities for diabetes and cardiovascular target discovery.
Finally, an important limitation of current cardiac organoids is the cells often do not express markers associated
with maturity, unlike native tissue, meaning that they may not be able to accurately replicate the full range of cardiac functions. Growth factors and cytokines have been identified to play essential roles during cardiac development and are necessary for cardiac tissue regeneration processes, therefore have been explored as key components of cardiac stem cell and organoid culture focusing on the maturation of the cardiac organoids. Cytokines such as Activin A, bone morphogenic protein 4 (BMP4), and vascular epithelial growth factor (VEGF) have all been found to contribute to cardiac lineage commitment when used in cardiac stem cell culture systems. In particular, Activin A and BMP4 have been associated with higher levels of myocyte markers in cardiac organoid cultures, whereas VEGF has been found to stimulate cardiomyocyte proliferation. This indicates the importance of selective growth factor and cytokine use in cardiac stem cell and organoid culture systems and the potential to push cardiac organoids to form more adult-like cell types.
Recent developments in cardiac research have enabled scientists to create three-dimensional organoids with atrial tissue, to provide more accurate models for studying atrial maturation processes. By supplementing these atrial organoids with growth factors, such as BMP9, cell maturity, and maturation were partially increased. There are, however, still some challenges that need to be overcome before atrial organoids can offer reliable, functional cardiac tissues and organs. Further research into controlling atrial organoid maturation at the genetic level will promote progress in this field. Moreover, further study into specializing atrial organoids using various techniques could yield meaningful results that have the potential to revolutionize cardiac treatments in the future. Increasing the complexity of cell-cell interactions through introducing additional neuronal and endothelial cells, and exploring the contribution from the extracellular matrix, as part of cardiomyocyte-containing cardiac organoids is being explored to improve the maturity and clinical relevance of these systems.
Cardiac organoid models and related 3D models such as spheroids are powerful tools that are poised to start to contribute to the early detection of drug, environmental toxin, and pollutants cardiac toxicity, and have applications in regenerative medicine. At Qkine we specialize in animal-free, high-purity, highly reproducible growth factors for commercial manufacture of organoid cultures and research use. Our team are happy to discuss collaborative or supply chain enhancement projects to scale-up and improve reproducibility in your organoid models.
Please contact us at proteins@qkine.com, or visit our organoid resource page for more information.
Quick answers
Which cell types are included in cardiac organoids?
The cell composition of cardiac organoids and related models is dependent on the complexity of the model. The main cell types in cardiac organoids are cardiomyocytes (CM). In many more complex models, endocardial (EC) and epicardial cells are present, representing the three main cardiac cell lineages.
How do you differentiate human pluripotent stem cells to cardiac cells?
Cardiac differentiation is generally considered to be induced by the activation of Wnt, Activin A, and BMP (usually BMP4) signaling to induce mesoderm, followed by Wnt-inhibition to generate cardiac mesoderm and subsequently cardiomyocytes. Cardiac differentiation is more straightforward than many iPSC differentiation relying primarily on bi-phasic modulation of Wnt signaling, first activation followed by inhibition.
Which growth factors are used in cardiac organoid culture?
Growth factors and cytokines used for cardiac organoids typically include Activin A, fibroblast growth factor-2 (FGF-2 or bFGF), BMP-4, vascular endothelial growth factor (VEGFA/VEGF-165), stem cell factor (SCF), and interleukin-6 (IL-6). These are important for the growth, differentiation, and function of cardiac cells. Additionally, other growth factors, such as insulin-like growth factor-1 (IGF-1) and transforming growth factor-beta (TGF-β1), are used in several protocols to promote the survival and differentiation of cardiac progenitor cells. Other BMP family members such as BMP-9 are used to promote maturation.
Recommended reading
1. Hofbauer, P., Jahnel, S. M. & Mendjan, S. In vitro models of the human heart. Development 148, dev199672 (2021). – https://journals.biologists.com/dev/article/148/16/dev199672/271916/In-vitro-models-of-the-human-heart
2. Kahn-Krell, A. et al. A three-dimensional culture system for generating cardiac spheroids composed of cardiomyocytes, endothelial cells, smooth-muscle cells, and cardiac fibroblasts derived from human induced-pluripotent stem cells. Front. Bioeng. Biotechnol. 10, 908848 (2022). – https://www.ncbi.nlm.nih.gov/pmc/articles/PMC9361017/
3. Williams, T.L., Colzani, M.T., Macrae, R.G.C. et al. Human embryonic stem cell-derived cardiomyocyte platform screens inhibitors of SARS-CoV-2 infection. Commun Biol 4, 926 (2021).